Whispers from the dark side: Confronting light new physics with NANOGrav data
Wolfram Ratzinger, Pedro Schwaller
SciPost Phys. 10, 047 (2021) · published 23 February 2021
- doi: 10.21468/SciPostPhys.10.2.047
- Submissions/Reports
-
Abstract
The NANOGrav collaboration has recently observed first evidence of a grav- itational wave background (GWB) in pulsar timing data. Here we explore the possibility that this GWB is due to new physics, and show that the signal can be well fit also with peaked spectra like the ones expected from phase transitions (PTs) or from the dynamics of axion like particles (ALPs) in the early universe. We find that a good fit to the data is obtained for a very strong PT at temperatures around 1 MeV to 10 MeV. For the ALP explanation the best fit is obtained for a decay constant of F ≈ 5 × 10^17 GeV and an axion mass of 2 × 10^−13 eV. We also illustrate the ability of PTAs to constrain the parameter space of these models, and obtain limits which are already comparable to other cosmological bounds.
TY - JOUR
PB - SciPost Foundation
DO - 10.21468/SciPostPhys.10.2.047
TI - Whispers from the dark side: Confronting light new physics with NANOGrav data
PY - 2021/02/23
UR - https://scipost.org/SciPostPhys.10.2.047
JF - SciPost Physics
JA - SciPost Phys.
VL - 10
IS - 2
SP - 047
A1 - Ratzinger, Wolfram
AU - Schwaller, Pedro
AB - The NANOGrav collaboration has recently observed first evidence of a grav- itational wave background (GWB) in pulsar timing data. Here we explore the possibility that this GWB is due to new physics, and show that the signal can be well fit also with peaked spectra like the ones expected from phase transitions (PTs) or from the dynamics of axion like particles (ALPs) in the early universe. We find that a good fit to the data is obtained for a very strong PT at temperatures around 1 MeV to 10 MeV. For the ALP explanation the best fit is obtained for a decay constant of F ≈ 5 × 10^17 GeV and an axion mass of 2 × 10^−13 eV. We also illustrate the ability of PTAs to constrain the parameter space of these models, and obtain limits which are already comparable to other cosmological bounds.
ER -
@Article{10.21468/SciPostPhys.10.2.047,
title={{Whispers from the dark side: Confronting light new physics with NANOGrav data}},
author={Wolfram Ratzinger and Pedro Schwaller},
journal={SciPost Phys.},
volume={10},
pages={047},
year={2021},
publisher={SciPost},
doi={10.21468/SciPostPhys.10.2.047},
url={https://scipost.org/10.21468/SciPostPhys.10.2.047},
}
Cited by 79
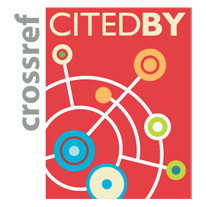
-
Gangopadhyay et al., Is the NANOGrav detection evidence of resonant particle creation during inflation?
Journal of High Energy Astrophysics 47, 100358 100358 (2025) [Crossref] -
Xue et al., Constraining Cosmological Phase Transitions with the Parkes Pulsar Timing Array
Phys. Rev. Lett. 127, 251303 (2021) [Crossref] -
Oikonomou, Implications of a scalar field interacting with the dark matter fluid on primordial gravitational waves
Phys. Rev. D 110, 023535 (2024) [Crossref] -
Lamb et al., Rapid refitting techniques for Bayesian spectral characterization of the gravitational wave background using pulsar timing arrays
Phys. Rev. D 108, 103019 (2023) [Crossref] -
Brandenburg et al., The scalar, vector, and tensor modes in gravitational wave turbulence simulations
Class. Quantum Grav. 38, 145002 (2021) [Crossref] -
Lee et al., Probing small-scale power spectra with pulsar timing arrays
J. High Energ. Phys. 2021, 28 (2021) [Crossref] -
Mansoori et al., PBHs and GWs from 𝕋2-inflation and NANOGrav 15-year data
J. Cosmol. Astropart. Phys. 2023, 067 (2023) [Crossref] -
Kitajima et al., Stochastic gravitational wave background from early dark energy
J. Cosmol. Astropart. Phys. 2023, 074 (2023) [Crossref] -
Yokoyama, Implication of pulsar timing array experiments on cosmological gravitational wave detection
AAPPS Bull. 31, 17 (2021) [Crossref] -
Domenech, Scalar Induced Gravitational Waves Review
Universe 7, 398 (2021) [Crossref] -
Li et al., Precision cosmology and the stiff-amplified gravitational-wave background from inflation: NANOGrav, Advanced LIGO-Virgo and the Hubble tension
J. Cosmol. Astropart. Phys. 2021, 024 (2021) [Crossref] -
Lewicki et al., Electroweak bubble wall expansion: gravitational waves and baryogenesis in Standard Model-like thermal plasma
J. High Energ. Phys. 2022, 17 (2022) [Crossref] -
Sun et al., Pulsar timing residual induced by wideband ultralight dark matter with spin 0,1,2
Phys. Rev. D 106, 066006 (2022) [Crossref] -
Papageorgiou et al., Axion dark matter from frictional misalignment
J. High Energ. Phys. 2023, 169 (2023) [Crossref] -
Ghosh et al., Did we hear the sound of the Universe boiling? Analysis using the full fluid velocity profiles and NANOGrav 15-year data
J. Cosmol. Astropart. Phys. 2024, 100 (2024) [Crossref] -
Gouttenoire, First-Order Phase Transition Interpretation of Pulsar Timing Array Signal Is Consistent with Solar-Mass Black Holes
Phys. Rev. Lett. 131, 171404 (2023) [Crossref] -
Barish et al., Impact of a midband gravitational wave experiment on detectability of cosmological stochastic gravitational wave backgrounds
Phys. Rev. D 103, 123541 (2021) [Crossref] -
Batell et al., Gravitational Waves from Nnaturalness
J. High Energ. Phys. 2024, 148 (2024) [Crossref] -
Afzal et al., The NANOGrav 15 yr Data Set: Search for Signals from New Physics
ApJL 951, L11 (2023) [Crossref] -
Chen et al., Search for the Gravitational-wave Background from Cosmic Strings with the Parkes Pulsar Timing Array Second Data Release
ApJ 936, 20 (2022) [Crossref] -
Li et al., Collider test of nano-Hertz gravitational waves from pulsar timing arrays
Phys. Rev. D 108, 055018 (2023) [Crossref] -
Chiang et al., Testing clockwork axion with gravitational waves
J. Cosmol. Astropart. Phys. 2021, 049 (2021) [Crossref] -
Domènech et al., NANOGrav hints on planet-mass primordial black holes
Sci. China Phys. Mech. Astron. 65, 230411 (2022) [Crossref] -
Madge et al., Audible axions with a booster: Stochastic gravitational waves from rotating ALPs
SciPost Phys. 12, 171 (2022) [Crossref] -
Kitajima et al., Gravitational waves from domain wall collapse, and application to nanohertz signals with QCD-coupled axions
Physics Letters B 851, 138586 138586 (2024) [Crossref] -
Arzoumanian et al., Searching for Gravitational Waves from Cosmological Phase Transitions with the NANOGrav 12.5-Year Dataset
Phys. Rev. Lett. 127, 251302 (2021) [Crossref] -
Zhang et al., Detecting new fundamental fields with pulsar timing arrays
Phys. Rev. D 108, 104069 (2023) [Crossref] -
Ferrer et al., Imprints of a supercooled phase transition in the gravitational wave spectrum from a cosmic string network
J. High Energ. Phys. 2023, 36 (2023) [Crossref] -
Freese et al., Gravitational wave spectrum of chain inflation
Phys. Rev. D 110, 103526 (2024) [Crossref] -
Lewicki et al., Gravitational waves from bubble collisions and fluid motion in strongly supercooled phase transitions
Eur. Phys. J. C 83, 109 (2023) [Crossref] -
Cheng et al., Detecting the stochastic gravitational wave background with the TianQin detector
Phys. Rev. D 106, 124027 (2022) [Crossref] -
Fujikura et al., NANOGrav signal from a dark conformal phase transition
Physics Letters B 846, 138203 138203 (2023) [Crossref] -
Dent et al., Sensitivity to dark sector scales from gravitational wave signatures
J. High Energ. Phys. 2022, 300 (2022) [Crossref] -
Borah et al., A first order dark SU(2)
D
phase transition with vector dark matter in the light of NANOGrav 12.5 yr data
J. Cosmol. Astropart. Phys. 2021, 039 (2021) [Crossref] -
Ramberg et al., One μ to rule them all: CMB spectral distortions can probe domain walls, cosmic strings and low scale phase transitions
J. Cosmol. Astropart. Phys. 2023, 039 (2023) [Crossref] -
Athron et al., Can Supercooled Phase Transitions Explain the Gravitational Wave Background Observed by Pulsar Timing Arrays?
Phys. Rev. Lett. 132, 221001 (2024) [Crossref] -
Li et al., NANOGrav signal from first-order confinement-deconfinement phase transition in different QCD-matter scenarios
Phys. Rev. D 104, 043510 (2021) [Crossref] -
Chigusa et al., Implications of gravitational waves for supersymmetric grand unification
Phys. Rev. D 104, 035031 (2021) [Crossref] -
Moore et al., Ultra-low-frequency gravitational waves from cosmological and astrophysical processes
Nat Astron 5, 1268 (2021) [Crossref] -
Borah et al., Gravitational waves from a dark
U(1)D
phase transition in light of NANOGrav 12.5 yr data
Phys. Rev. D 104, 063501 (2021) [Crossref] -
Liu, Distinguishing the nanohertz gravitational-wave sources by the observations of compact dark matter subhalos
Phys. Rev. D 108, 123544 (2023) [Crossref] -
Banerjee et al., Gravitational wave echo of relaxion trapping
Phys. Rev. D 104, 055026 (2021) [Crossref] -
Bian et al., The Gravitational-wave physics II: Progress
Sci. China Phys. Mech. Astron. 64, 120401 (2021) [Crossref] -
Freese et al., Have pulsar timing arrays detected the hot big bang: Gravitational waves from strong first order phase transitions in the early Universe
Phys. Rev. D 106, 103523 (2022) [Crossref] -
Ge, Stochastic gravitational wave background: birth from string-wall death
J. Cosmol. Astropart. Phys. 2024, 064 (2024) [Crossref] -
Lewicki et al., Bubble dynamics in fluids with
N
-body simulations
Phys. Rev. D 106, 103501 (2022) [Crossref] -
Barir et al., Gravitational waves from incomplete inflationary phase transitions
Phys. Rev. D 108, 115016 (2023) [Crossref] -
Lewicki et al., Escape from supercooling with or without bubbles: gravitational wave signatures
Eur. Phys. J. C 81, 857 (2021) [Crossref] -
Zheng et al., Quantitative analysis of the gravitational wave spectrum sourced from a first-order chiral phase transition of QCD
Phys. Rev. D 111, L021303 (2025) [Crossref] -
Depta et al., Signals of merging supermassive black holes in pulsar timing arrays
Phys. Rev. Research 7, 013196 (2025) [Crossref] -
Maiorano et al., Principles of Gravitational-Wave Detection with Pulsar Timing Arrays
Symmetry 13, 2418 (2021) [Crossref] -
Han et al., Self-interacting dark matter implied by nano-Hertz gravitational waves
Phys. Rev. D 109, 115025 (2024) [Crossref] -
Samanta et al., Gravitational wave complementarity and impact of NANOGrav data on gravitational leptogenesis
J. High Energ. Phys. 2021, 211 (2021) [Crossref] -
Megías et al., Pulsar timing array stochastic background from light Kaluza-Klein resonances
Phys. Rev. D 108, 095017 (2023) [Crossref] -
Yi et al., NANOGrav signal and LIGO-Virgo primordial black holes from the Higgs field
J. Cosmol. Astropart. Phys. 2022, 046 (2022) [Crossref] -
Blas et al., Bridging the
μHz
Gap in the Gravitational-Wave Landscape with Binary Resonances
Phys. Rev. Lett. 128, 101103 (2022) [Crossref] -
Roper Pol et al., Gravitational wave signal from primordial magnetic fields in the Pulsar Timing Array frequency band
Phys. Rev. D 105, 123502 (2022) [Crossref] -
Bai et al., Cosmological constraints on first-order phase transitions
Phys. Rev. D 105, 095015 (2022) [Crossref] -
Oikonomou, Flat energy spectrum of primordial gravitational waves versus peaks and the NANOGrav 2023 observation
Phys. Rev. D 108, 043516 (2023) [Crossref] -
Odintsov et al., The necessity of multi-band observations of the stochastic gravitational wave background
Physics of the Dark Universe 46, 101562 101562 (2024) [Crossref] -
Chen et al., Asymmetric dark matter with a spontaneously broken
U(1)′
: Self-interaction and gravitational waves
Phys. Rev. D 107, 095027 (2023) [Crossref] -
Madge et al., Primordial gravitational waves in the nano-Hertz regime and PTA data — towards solving the GW inverse problem
J. High Energ. Phys. 2023, 171 (2023) [Crossref] -
Ahmadvand et al., Heavy QCD axion model in light of pulsar timing arrays
Phys. Rev. D 108, 115020 (2023) [Crossref] -
Gouttenoire et al., Domain wall interpretation of the PTA signal confronting black hole overproduction
Phys. Rev. D 110, L061306 (2024) [Crossref] -
Kitajima et al., Nonthermally trapped inflation by tachyonic dark photon production
Phys. Rev. D 105, 103011 (2022) [Crossref] -
Bringmann et al., Does NANOGrav observe a dark sector phase transition?
J. Cosmol. Astropart. Phys. 2023, 053 (2023) [Crossref] -
Benetti et al., Primordial gravitational waves from NANOGrav: A broken power-law approach
Phys. Rev. D 105, 043520 (2022) [Crossref] -
Sharma, Constraining models of inflationary magnetogenesis with NANOGrav data
Phys. Rev. D 105, L041302 (2022) [Crossref] -
Tasinato, Gravitational wave nonlinearities and pulsar-timing array angular correlations
Phys. Rev. D 105, 083506 (2022) [Crossref] -
Vagnozzi, Inflationary interpretation of the stochastic gravitational wave background signal detected by pulsar timing array experiments
Journal of High Energy Astrophysics 39, 81 (2023) [Crossref] -
Ünal et al., Axion-gauge dynamics during inflation as the origin of pulsar timing array signals and primordial black holes
Physics Letters B 856, 138873 138873 (2024) [Crossref] -
Eichhorn et al., From quantum gravity to gravitational waves through cosmic strings
Phys. Rev. D 109, 026013 (2024) [Crossref] -
Freese et al., Dark matter and gravitational waves from a dark big bang
Phys. Rev. D 107, 083522 (2023) [Crossref] -
Gould et al., On the perturbative expansion at high temperature and implications for cosmological phase transitions
J. High Energ. Phys. 2021, 69 (2021) [Crossref] -
Sójka et al., Radiative symmetry breaking from the on-shell perspective
Physics Letters B 860, 139155 139155 (2025) [Crossref] -
Winkler et al., Origin of the stochastic gravitational wave background: First-order phase transition versus black hole mergers
Phys. Rev. D 111, 083509 (2025) [Crossref] -
Oikonomou et al., Primordial cosmology of an emergent-like universe from modified gravity: Reconstruction and phenomenology optimization with a genetic algorithm
Int. J. Mod. Phys. D 33, 2350114 (2024) [Crossref] -
Bian et al., Gravitational wave sources for pulsar timing arrays
Phys. Rev. D 109, L101301 (2024) [Crossref] -
Ratzinger et al., Gravitational waves from an axion-dark photon system: A lattice study
SciPost Phys. 11, 001 (2021) [Crossref]